A Beginners Guide to Phage Research
- Dave Black
- Nov 1, 2023
- 6 min read
The ABAtE (Active Bacteriophages for American FoulBrood Eradication) project born at Massey University has gone from strength to strength at University of Canterbury, now part of a funded five-year programme to harness bacteriophages and create a new bioindustry serving primary industries in New Zealand. This ambitious project still has apiculture, and American Foulbrood (AFB) control, at its heart. Here we look at what bacteriophages are, what they can do, and what’s involved in making them a practical and sustainable option for disease control.
Meet the bacteriophage
‘Phage’ is a colloquial contraction of ‘bacteriophage’, literally, a devourer (eater) of bacteria. Beekeepers are not alone in having an interest in things that will eat bacteria, but the whole topic is – to put it mildly – intimidating. This ‘thing’ that eats bacteria is a virus, a ‘thing’ that exists in the grey zone you didn’t know existed between the black and white of ‘dead’ or ‘alive’. To this day, viruses render biology, philosophy, and Wikipedia unable to define definitely what the property of being alive means. And they have inscrutable names, like Sphaerolipoviridae and Matshushitaviridae.

That’s not all. Even in the highest most pristine environments viruses rain down depositing between about one quarter of a billion and seven billion virus particles on every square metre. Mostly from marine sources, there are estimated to be 1030 virus particles just in the world’s oceans, they outnumber all the other biological entities on earth combined[i]. The human microbiome is thought to contain 30-40 trillion (1012) bacteria, roughly on par with the number of human cells. The human ‘virome’, the virus particles that also live on or within us, is expected to have ten times the number of bacterial cells. It’s not unlike trying to guess the amount of ‘dark matter’ in the universe, the numbers are beyond comprehension.
The astonishing genetic and physical diversity of bacteriophages defies belief, and certainly taxonomic classification systems. The concept of a ‘species’ is not specific enough to be used to describe the similarities and relationships between them. Identical, or nearly identical, virus particles can be found in completely different environments, and yet the same environment hosts totally different types of particle, while ‘phages can be so particular to their host cells that they have been used in diagnostic tests to identify strains of bacteria. This apparent chaos and ubiquity is most likely to be because of their global atmospheric persistence and dispersal; the only limitation is probably the bio-geographic limits on the distribution of their hosts. Some writers imagine viruses and bacteria as a vast global atmospheric ‘soup’ of shared
genetic material.
A History of Phage Finds
The first ‘phages that attack the bacteria that causes AFB were recorded in the 1950s, first in Russia (1953) and, for the English-speaking world, by T.A. Gochnauer at the Canadian Department of Agriculture in 1955. It was a discovery not thought particularly significant at the time and it would be 60 years before we began to isolate and sequence the genome of more, just as antibiotic resistance was becoming a concern. By 2020 48 types from several countries had been sequenced and published[ii]. The ABAtE Project at the University of Canterbury have added another 26[iii].
The last 20 years have produced a modest surge in the effort to understand and use ‘phages to treat all sorts of bacterial diseases, and a handful of products have regulatory approvals for treating, for example, Fireblight[iv]. Another, not yet with any approval but to be part of the Canterbury project, is kiwifruit PSA. Some other Pseudomonas bacterial diseases have approved ‘phage products, but these are not yet widely used. Phage ‘cocktails’ have also been recognised as potentially a useful green technology for combating food-borne pathogenic and spoilage bacteria for the food industry.
Non-GM biocontrol
Bacteriophage controls have some quite attractive advantages as disease control products. They can be highly specific to a given bacterial species or strain, found everywhere and clearly have no ‘off-target’ effects; plants and animals are always exposed to them already. They are easy to isolate, can be applied in low concentrations, penetrate bacterial biofilms, and accumulate or multiply exponentially where they are needed, for only as long as the target pest is present. They have no residue. ‘Phages can also adapt and mutate to overcome resistance mechanisms bacteria may develop.
A successful ‘phage hunt has many things to think about. The first stage of the ABAtE project was to catalogue the strains of AFB bacteria we have in New Zealand. You have to carefully identify the bacterial host so you get the right ‘phage(s). Bacteria can, and do, develop resistance to ‘phages. Developing ‘cocktails’ incorporating several strains is a clever mitigation, but it isn’t always easy to find enough compatible strains. ‘Phages can be quite fragile and sensitive to radiation (light), heat, and ion concentration. That’s not ideal in an agricultural setting with potentially high (or low) temperatures, high exposure to ultraviolet radiation and agricultural chemicals, desiccation, and fluctuating soil moisture or pH. Not only are ‘phages in competition with bacteria, they can be in competition with each other too, and there appear to be lots of ‘phages simply not suitable for one reason or another. For example, they might induce the bacteria to make a toxin.
The potential pool of therapeutic ‘phages can notionally be grouped in two: ‘phages can be ‘virulent’ or ‘temperate’. Virulent ‘phages enter a host cell and immediately start to use the cell to make copies of themselves, after which they lyse (break apart) the host cell to release the new ‘phages. Temperate ‘phages are sneakier, entering the cell and integrating their genetic material into the host’s. The infected bacterium is called a lysogen, and the integrated ‘phage is called a prophage. The intertwined evolutionary success, and virulence, of this combined entry can be very different from the original bacterium. At some future point the bacterial cell will be destroyed and the prophages released as ‘phages.
Virulent ‘phages are therefore also described as ‘lytic’ (they lyse things), and temperate ‘phages are also described as ‘lysogenic’ (they make lysogens). Complicating that description (nothing about ‘phages is simple) some ‘phages operate both life-cycles, switching from one to the other depending on circumstances we don’t understand. I wouldn’t have brought that up, except that all the AFB ‘phages so far known, including the New Zealand ones, are temperate (lysogenic) in some circumstances and virulent (lytic) in others. We know from the ABAtE’s study the AFB bacteria contain prophages, and we know the bacteriophages produce a protein (integrase) that helps to join the ‘phage genome to the bacteria’s genome. That all matters because it limits the pool of ‘phages that can be used, if the wrong one is used our problem gets worse not better. It’s essential that every potentially useful ‘phage is carefully examined and tested. In the case of kiwifruit, most of the candidate ‘phages are lytic, so these are the ones selected for use, which perhaps simplifies the matter.
Commercial Solutions
The regulations that govern the use of ‘phage ‘therapy’ can be challenging, if they exist at all. New Zealand biosecurity laws preclude the import of non-native 'phages for domestic release so we have to find local ones. Given the omnipresence of ‘phages described earlier, that seems silly. Given also the specificity of the bacterium to bacteriophage relationship, it probably doesn’t change anything – we would have to find local ones anyway. As with anything new the rules are not properly developed, and not coordinated between jurisdictions.
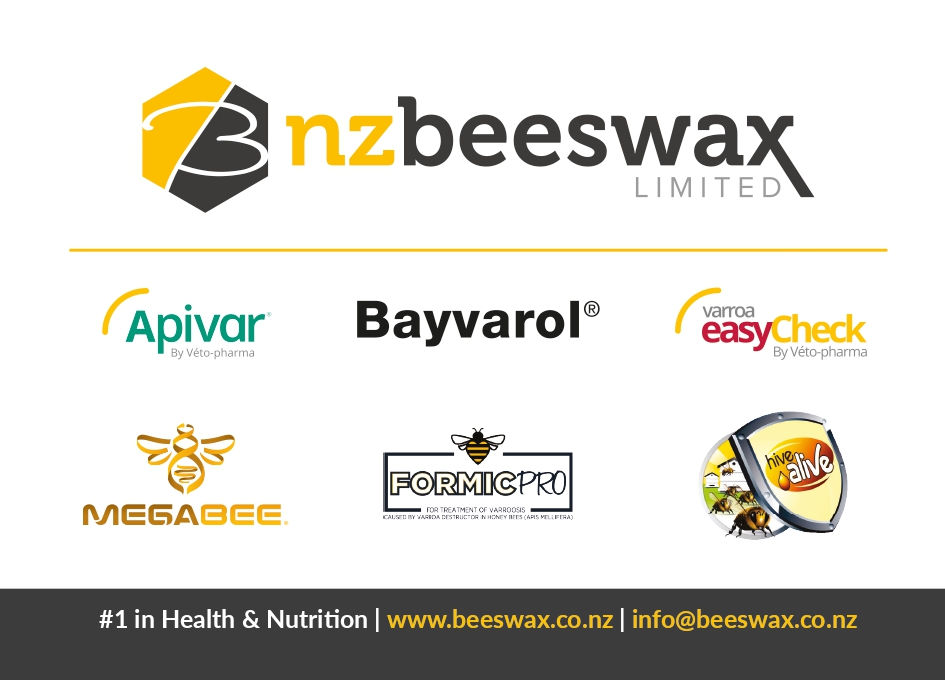
The big global organizations approving 'phage cocktails used in agriculture are the European Food Safety Authority (EFSA) and the US Food and Drug Administration (FDA). One particular problem with 'phage-mediated biocontrol is that phage mixtures/cocktails need to be revised all the time to lyse as many newly emerging strains of the target bacterium as possible. However, EU regulations, but not US regulations, require that any change to one of the phage cocktail’s components would need re-registering and adhere to GMP (Good Manufacturing Practice), which is time consuming and expensive.
An issue related to the regulatory environment that will already constrain the production of ‘phage-based treatments are the prevailing economic circumstances[v]. A conventional medicine like the latest antibiotic is developed once and sold, and can be neatly ‘packaged’ with its intellectual property (IP) rights. ‘Phage therapies are not like that. We should expect that they are sourced, characterised, developed and constantly redeveloped and recombined. How then do we engineer a financially sustainable process, and who’s IP is used where? (see Figure 1.)

The work in Canterbury is awesome, serious new science and enormously ambitious. That it continues to engage with its ‘citizens’ too is a credit to the team. If New Zealand is going to convert research success into an industry providing economic and social benefit from ‘phage therapies – and that is the whole point of the programme – that multi-disciplinary engagement will be an essential component.
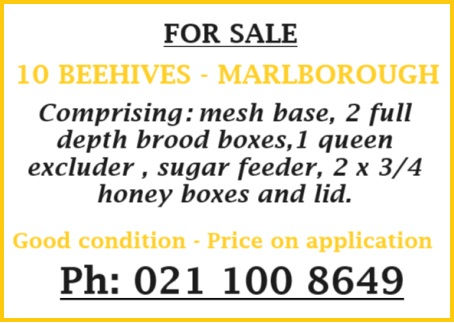
References [i] Curtis A. Suttle Viruses in the sea Nature, Vol 437,15 September 2005, doi:10.1038/nature04160 [ii] Casey Stamereilers et al, Genomic Analysis of 48 Paenibacillus larvae Bacteriophages, Viruses 2018, 10, 377; doi:10.3390/v10070377 [iii] Kok DN, Zhou D, Tsourkas PK, Hendrickson HL. Paenibacillus larvae and their phages; a community science approach to discovery and initial testing of prophylactic phage cocktails against American Foulbrood in New Zealand. Microbiome Res Rep 2023;2:30. https://dx.doi.org/10.20517/mrr.2023.16 [iv] Carla Pereira, Pedro Costa, Larindja Pinheiro, Victor M. Balcão, Adelaide Almeida, Kiwifruit bacterial canker: an integrative view focused on biocontrol strategies Planta (2021) 253:49 https://doi.org/10.1007/s00425-020-03549-1 [v] Jean-Paul Pirnay et al. The Phage Therapy Paradigm: Prêt-à-Porter or Sur-mesure? Pharm Res (2011) 28:934–937 DOI 10.1007/s11095-010-0313-5 https://www.researchgate.net/publication/47730148 See also Jean-Paul Pirnay et al, The Magistral Phage, Viruses (2018), 10, 64; doi:10.3390/v10020064
Comments